If you only have a few seconds:
Hypoventilation training consists of performing phases of effort while holding your breath, intermittently. This training method delays muscle fatigue due to lactate accumulation. It could also increase endurance performance and improve psychocognitive resilience to fatigue and breathlessness.
…if you have a few minutes, read on…
Foreword:
The background information presented in this post is taken from X. Woorons, Hypoventilation Training, Push Your Limits! available here.
Hypoventilation training is an important part of the breathing tools offered by the Oxygen Advantage and REBO2T methods, which I teach. For more information, I recommend Patrick McKeown’s The Breathing Cure (sponsored link) and, if you read French, Yvan Cam’s La Maîtrise du Souffle.
N.B.: During hypoventilation training, heart rate and blood pressure increase, compared to normal training. Hypoventilation training is therefore not recommended in cases of high blood pressure. In such cases, the advice of a doctor is recommended. In addition, hypoventilation training can cause headaches, depending on the intensity of the exercises and from one person to another 1,2.
Ventilation
What the average person calls breathing is what physiologists call ventilation. This term describes the action of inflating and deflating the lungs. Ventilation is therefore the stage where respiration regulates not only the supply of O2 from the external environment (the atmosphere) to the internal environment (the blood and then the tissues and cells), but also the evacuation of CO2 from the internal environment to the external environment. The speed and amplitude at which we ventilate are therefore parameters that have a direct impact on the O2 and CO2 levels in our bodies. These levels must be within a window of values that allow the body to adapt its metabolism to the energy demand: to ensure the supply of O2 to the cells in order to produce energy in the mitochondria and to evacuate the excess CO2 in order to avoid breathlessness. Correct ventilation, consistent with homeostasis, will allow the body to maintain a SaO2 between 96% and 99% and a PCO2 of ≈40mmHg over a wide window of metabolic demand. But it is possible to hyperventilate or hypoventilate. Respiratory homeostasis is then compromised and the consequences may be more or less severe for the body. These conditions can be acute or chronic, pathological, involuntary or voluntary. The purpose of this post is not to present an exhaustive description of these disorders but a voluntary and transient use of hypoventilation: hypoventilation training.
Training with hypoventilation
Hypoventilation is ventilation that is insufficient to ensure the supply of air to the body. The balance of respiratory gases is then disturbed: in the pulmonary alveoli as well as in the blood plasma, O2 decreases and CO2 increases, leading to a hypercapnic hypoxemic situation which is more or less marked according to the severity of the hypoventilation. Hypoventilation training thus consists of inducing such an imbalance by voluntarily reducing ventilation. Technically, this is done by consciously decreasing the respiratory frequency and/or amplitude. But in practice, it consists in alternating phases of respiratory hold with phases of recovery by breathing normally. In this post about training, this alternation is carried out during a physical effort (running, cycling, swimming…)
Historically, Eastern European athletes, including the famous Zatopek, were the first to experiment with breath-holding during exercise in the 1950s. In the West, swimming and running coaches then used similar techniques. But it was not until the 1990s and especially the 2000s that scientific study protocols were put in place to rigorously investigate these techniques. A decisive step was taken at the University of Paris 13 in the 2000s. Indeed, a protocol of breath retention at the end of exhalation was proposed, instead of full-lung retention after inspiration, as it was done before. This protocol allows to observe rapid physiological changes. On the cardiovascular system, the heart rate slows down during breath hold and increases when breathing resumes, impacting blood pressure. Regarding the metabolic state, hypoventilation training induces severe hypoxemia (SaO2 easily drops from ≈97% to ≈87%), hypercapnia (PCO2 at 50mmHg instead of 40mmHg) and acidosis 1.
Why training in hypoventilation?
This breathing sequence, by retention of the breath at the end of expiration (and not after inspiration), seems crucial to observe this acidosis during intermittent hypoxemia. It is both a respiratory acidosis, due to the increase in PCO2, and a metabolic acidosis, since hypoxemia leads to anaerobic glycolysis and lactate production. This increase of lactatemia is only observe using the post-exhale breath hold protocol, not when performing post-inhale breath hold. Moreover, it is very likely that hypoxia, hypercapnia and acidification are even more important in muscle tissue than in blood 1. This very combination of hypoxia, hypercapnia and acidosis is crucial to induce a metabolic stress which triggers buffering capacity of muscle cells. Moreover, these effects are all the more important that the longer the breath hold is 3. It appears that this type of training improves the use of O2 by the muscles (myoglobin content, mitochondria, capillary density, etc.) but also the response of the muscles to anaerobic-induced acidification (increase in the expression of carbonic anhydrase and monocarboxylate transporter 1) 1. Muscle reoxygenation during recovery phases would also be improved 4. By stimulating the activation of buffer compounds (phosphate, proteins…), these exercises train the muscles to neutralise the acidification linked to the accumulation of lactate, thus delaying fatigue 1. The transmembrane flow of potassium would also play a role and would be involved in the lesser muscle fatigue observed 4.
Finally, the retention of the breath allows us to observe a significant metabolic response, through the increase in lactatemia, even though the effort provided is moderate. This could allow the athlete to maintain this anaerobic workload when he or she is unable to train at maximum power due to injury 1,5.
An equivalent to a stay at altitude? A priori no
The scientific literature on hypoventilation training systematically reports the haemoglobin desaturation that occurs during these sessions. Typically, SaO2 drops to ≈87% (instead of the regular 96-99%), which corresponds to a desaturation that can be observed at altitudes above 2000m, due to the rarefaction of atmospheric air and O2 in particular 1. Thanks to a recently established mathematical model, it is possible to establish a relationship between SaO2, VO2 during effort (in % of VO2max) and the altitude level 6. It is then tempting to make a shortcut and equate hypoventilation training with a stay at altitude. The main benefit sought during the latter is the increase in the synthesis of red blood cells, erythropoiesis. Indeed, hypoxia leads to a physiological response: the overexpression of a transcription factor called HIF (Hypoxia Inducible Factor), which will in turn stimulate the synthesis of erythropoietin (EPO). The latter finally stimulates the production of red blood cells in the bone marrow (more precisely of their precursors – reticulocytes – which will then mature into red blood cells). More red blood cells means more convoys of haemoglobin, the main O2 transporting protein. Therefore, more red blood cells should guarantee a better transport of O2 to the muscles. It is therefore easy to understand why high-level athletes are interested in spending time at altitude. But these stays require time and resources. Moreover, training at altitude does not allow for the same intensity of training as at sea level. There is therefore a risk of “de-training” during prolonged stays at altitude, as well as a risk of injury. In short, having the benefits of staying at altitude without the disadvantages is the ideal situation. This is technically feasible by injecting EPO, but it contravenes sporting ethics and anti-doping regulations. Could hypoventilation training therefore be a realistic alternative?
To date, studies have not established an improvement in the delivery of O2 to the muscles by hypoventilation training. Despite the observed increase in HIF and EPO, red blood cells do not increase. Hypoventilation training exposes the athlete to hypoxia intermittently, over durations that are a priori too short to observe an effect on blood composition, at least by the protocols tested to date 7.
Generally speaking, this opens the question of the hypoxic dose necessary to increase red blood cell synthesis. Since the hypoventilation training protocols studied to date are not sufficient, let’s look at other forms of hypoxia exposure. The recent study by Park and colleagues reports data from hypoxic chamber training. This training is varied and consists of exposing athletes to low O2 atmospheres (12-15% instead of 21%) for several one or two hour sessions per week for 10 days to 7 weeks. Some studies report an increase in red blood cells, others only in their precursors, and others only in EPO 8. Despite the apparent lack of consensus, this approach of intermittent exposure to hypoxia seems to have potential. But it is also quite cumbersome to implement for most sportsmen and women. So let’s look at free diving. The latter is indeed an obvious form of hypoxia exposure that does not require heavy equipment. In addition, high level freedivers have higher than average haematocrit levels. It is therefore interesting to understand the respective parts of genetic predisposition and adaptation by exposure to hypoxia in these athletes. A regime of 10 maximal apneas per day for 12 days resulted in a 15% increase in reticulocytes, the precursors of red blood cells, in novices 9. Also in novices, at the end of a 6-week training programme (10 dynamic apneas per session, 4 sessions per week), an increase of 28% in reticulocytes was observed but no change in mature red blood cells 10. These programmes therefore make it possible to trigger the synthesis of red blood cell precursors but these do not mature. It is possible that these programmes are simply too short in duration.
In order to explore the question of the hypoxic dose necessary for the synthesis of red blood cells and the increase in haematocrit, we can also look at obstructive sleep apnoea syndrome. Indeed, this medical condition, which is of course undesirable for anyone, particularly in the context of athletic preparation, nevertheless represents a real exposure to intermittent hypoxic stress. A recent meta-review indeed shows an increase in haematocrit in people with obstructive sleep apnoea. But this increase, even if statistically significant, is modest and is not considered to be a signature of abnormally high erythropoiesis, even in severely affected individuals with more than 30 hypopnea episodes per hour of sleep 11.
In short, science has not revealed the formula for stimulating red blood cell synthesis while living at low altitude and without a hypoxic chamber, just by breath-holding exercises. In the end, current hypoventilation training protocols can simulate altitude training but not altitude acclimatisation.
Training for endurance or speed?
The main benefit of hypoventilation training, which has been scientifically confirmed to date, concerns the improved resilience of the muscles to acidosis. This acidosis occurs rapidly during middle-distance events or sprint repetitions, where anaerobic metabolism predominates. Thus, such training makes it possible to increase one’s capacity to perform sprints or to increase one’s performance in short, intense events, from about thirty seconds to a few minutes, in various contexts (cycling, rugby, basketball, swimming, running) 1,4,12–14. A particularly striking example of these performance improvements is that obtained in professional rugby players. In just four weeks, these top athletes increased their performance in the sprint repetition test by 64% (compared to 9% in the control group) 13.
On the other hand, at present, studies have not reached a consensus on possible benefits in endurance sports. However, depending on the route, the gradient or even the running strategy, the accumulation of lactate in the muscles is more or less important. The regulation of the acid-base balance is typically threatened when the intensity increases above VO2max, which can happen in certain phases of the race. It is therefore possible to speculate that such training allows the athlete to be more resilient in relation to the phases of racing that generate acidosis, even in the overall context of endurance racing: managing a uphill section, passing a competitor, particularly at the end of the race when exhaustion is imminent. Furthermore, hypoventilation training, by working on the repetition of apneas, would allow the athlete to reduce his sensitivity to CO2. This reduction has the direct effect of delaying the sensation of breathlessness and favours better O2 transport via the Bohr effecta 2. Finally, from a more subjective point of view, this type of training includes a mental component consisting of putting oneself in situations of suffocation. It can be speculated that this practice may provide more mental resilience during the race 1.
But let’s go back to biological considerations. Some studies suggest that hypoxia training protocols may actually improve VO2max. In particular, 6 week programmes of intermittent hypoxia training at high intensity (90% of VO2max, 90-95% of maximum heart rate) have shown an improvement in VO2max 8,15. Could this hypoxic dose be achieved by hypoventilation? Furthermore, it has been shown that, during hypoventilation training, at the time of breath holds, the heart rate was reduced. This physiological response, which is a mechanism for conserving oxygen by reducing the workload of the heart, is partially compensated for by an increase in systolic ejection volume 16. The authors of this study hypothesise that such training could promote greater ventricular compliance. It is thus possible to speculate that such an adaptation could be beneficial for long-distance races. Another study has shown benefits of hypoventilation training on running endurance when trained on a bicycle 17. In this study, six sessions over a three-week period (on a bicycle) improved scores on the Yo-Yo Intermittent Recovery Level 1 endurance test by 32%. This test was also shown to correlate with VO2max. This improvement could be linked to the adaptation of cardiac activity mentioned above. It should also be noted that this study raises the important question of specific training: to prepare for running, is it better to train in hypoventilation by running or by cycling? Cautiously, I would answer: both. In fact, the specific training (running) will contribute to the adaptation of the muscles specific to running to manage acidification. Hypoventilation training on a bike, in addition to offering a welcome alternative to limit repetitive impacts, would favour the effects on endurance.
Conclusion
Studies on hypoventilation training are accumulating and seem to converge towards a consensus on their benefits for intense and short duration events, whether continuous or intermittent (repetitions of sprints). This type of training would allow the body to better manage metabolic and respiratory acidosis. As far as endurance events are concerned, research has not completely settled the issue. It is true that the current protocols do not seem to generate a hypoxic dose large enough to stimulate the synthesis of red blood cells, as during acclimatisation at altitude. However, a better resilience to acidosis is not without interest for an endurance athlete and this type of training could help to build mental strength in the face of breathlessness and fatigue. Finally, it is not excluded that this type of training can increase VO2max, and therefore have a benefit on endurance. Future studies may allow us to understand how to reach the hypoxic dose sufficient to increase one’s red blood cell count in the long term by practicing breath-holding. I look forward to reading more studies on this subject.
In the meantime, hypoventilation training seems to me a great tool to add to the ones I discussed previously in the context of running (here and here).
🔥❄️🧠✌️
Sébastien.
Acknowledgements: Big thanks to XW, PhD, and PMK for their help in improving the manuscript.
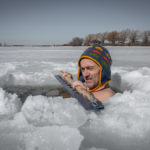
Sébastien Zappa, PhD
Oxygen Advantage Master InstructorREBO2T – Practitioner
Wim Hof Method Instructor Level 2
ELDOA Practitioner Level 2
Overall Breathing & Cold Geek, Homo cryopulmosapiens…
Happy to coach you since 2018
a When the CO2 content in the blood increases, this is called hypercapnia. The amount of CO2 in the blood determines the pH of the blood. Hypercapnia therefore changes the blood pH by making it more acidic. The chemical bond between hemoglobin and O2 in oxyhemoglobin is pH dependent. The more acidic the pH, the weaker this bond and the easier it is to release O2 to the tissues. This is the Bohr effect.
References :
1. Woorons, X. Hypoventilation training, push your limits! (ARPEH, 2014).
2. Harbour, E., Stöggl, T., Schwameder, H. & Finkenzeller, T. Breath Tools: A Synthesis of Evidence-Based Breathing Strategies to Enhance Human Running. Front Physiol 13, 813243 (2022).
3. Woorons, X., Billaut, F. & Lamberto, C. Running exercise with end-expiratory breath holding up to the breaking point induces large and early fall in muscle oxygenation. Eur J Appl Physiol 121, 3515–3525 (2021).
4. Lapointe, J., Paradis-Deschênes, P., Woorons, X., Lemaître, F. & Billaut, F. Impact of Hypoventilation Training on Muscle Oxygenation, Myoelectrical Changes, Systemic [K+], and Repeated-Sprint Ability in Basketball Players. Frontiers Sports Active Living 2, 29 (2020).
5. Guimard, A. et al. Exponential Relationship Between Maximal Apnea Duration and Exercise Intensity in Non-apnea Trained Individuals. Front Physiol 12, 815824 (2022).
6. Woorons, X. & Richalet, J. P. Modelling the relationships between arterial oxygen saturation, exercise intensity and the level of aerobic performance in acute hypoxia. Eur J Appl Physiol 121, 1993–2003 (2021).
7. Albertus-Cámara, I., Ferrer-López, V. & Martínez-González-Moro, I. The Effect of Normobaric Hypoxia in Middle- and/or Long-Distance Runners: Systematic Review. Biology 11, 689 (2022).
8. Park, H.-Y., Jung, W.-S., Kim, S.-W., Kim, J. & Lim, K. Effects of Interval Training Under Hypoxia on Hematological Parameters, Hemodynamic Function, and Endurance Exercise Performance in Amateur Female Runners in Korea. Front Physiol 13, 919008 (2022).
9. Engan, H., Richardson, M. X., Lodin‐Sundström, A., Beekvelt, M. & Schagatay, E. Effects of two weeks of daily apnea training on diving response, spleen contraction, and erythropoiesis in novel subjects. Scand J Med Sci Spor 23, 340–348 (2013).
10. Elia, A., Barlow, M. J., Wilson, O. J. & O’Hara, J. P. Six weeks of dynamic apnoeic training stimulates erythropoiesis but does not increase splenic volume. Eur J Appl Physiol 121, 827–838 (2021).
11. Rha, M. et al. Is obstructive sleep apnea associated with erythrocytosis? A systematic review and meta‐analysis. Laryngoscope Investigative Otolaryngology 7, 627–635 (2022).
12. Woorons, X., Mucci, P., Richalet, J. P. & Pichon, A. Hypoventilation Training at Supramaximal Intensity Improves Swimming Performance. Medicine Sci Sports Exerc 48, 1119–1128 (2016).
13. Fornasier-Santos, C., Millet, G. P. & Woorons, X. Repeated-sprint training in hypoxia induced by voluntary hypoventilation improves running repeated-sprint ability in rugby players. Eur J Sport Sci 18, 1–9 (2018).
14. Woorons, X., Millet, G. P. & Mucci, P. Physiological adaptations to repeated sprint training in hypoxia induced by voluntary hypoventilation at low lung volume. Eur J Appl Physiol 119, 1959–1970 (2019).
15. Dufour, S. P. et al. Exercise training in normobaric hypoxia in endurance runners. I. Improvement in aerobic performance capacity. J Appl Physiol 100, 1238–1248 (2006).
16. Woorons, X., Lemaitre, F., Claessen, G., Woorons, C. & Vandewalle, H. Exercise with End-expiratory Breath Holding Induces Large Increase in Stroke Volume. Int J Sports Med 42, 56–65 (2020).
17. Woorons, X., Billaut, F. & Vandewalle, H. Transferable Benefits of Cycle Hypoventilation Training for Run-Based Performance in Team-Sport Athletes. Int J Sport Physiol 15, 1103–1108 (2020).